In 1915 Albert Einstein published a geometrical theory of gravitation: The General Theory of Relativity. He presented a fundamentally new description of gravity in the sense that the relative acceleration of particles is not viewed as a consequence of gravitational forces but results from the curvature of the spacetime in which the particles are moving. As long as no non-gravitational forces act on a particle, it is always moving on a ``straight line''. If we consider curved manifolds there is still a concept of straight lines which are called geodesics , but these will not necessarily have the properties we intuitively associate with straight lines from our experience in flat Euclidean geometry. It is, for example, a well known fact that two distinct straight lines in 2-dimensional flat geometry will intersect each other exactly once unless they are parallel in which case they do not intersect each other at all. These ideas result from the fifth Euclidean postulate of geometry which plays a special role in the formulation of geometry.
It is a well known fact that one needs to impose it separately from the first four Euclidean postulates in order to obtain flat Euclidean geometry. It was not realised until the work of Gauss, Lobachevsky, Bolyai and Riemann in the 19th century that the omission of the fifth postulate leads to an entirely new class of non-Euclidean geometries in curved manifolds. A fundamental feature of non-Euclidean geometry is that straight lines in curved manifolds can intersect each other more than once and correspondingly diverge from and converge towards each other several times.
In order to illustrate how these properties give rise to effects we commonly associate with forces such as gravitation, we consider two observers on the earth's surface, say one in Southampton, UK, and one in Thessaloniki, Greece. We assume that these two observers start moving due south in ``straight lines'' as for example guided by an idealised compass exactly pointing towards the south pole. If we follow their separate paths we will discover exactly the ideas outlined above. As long as both observers are in the northern hemisphere the proper distance between them will increase and reach a maximum when they reach the equator. From then on they will gradually approach each other and their paths will inevitably cross at the south pole.
![[Geodesics]](Images/Soton-Thess2.gif)
In the framework of Newtonian physics the observers will attribute the relative acceleration of their positions to the action of a force. It is clear, however, that no force is acting in the east-west direction on either observer at any stage of their journey. In a geometric description the relative movement of the observers finds a qualitatively new interpretation in terms of the curvature of the manifold they are moving in, the curvature of the earth's surface. With the development of general relativity Einstein provided the exact mathematical foundation for applying these ideas to the forces of gravitation in 4-dimensional spacetime.
One may ask why such a geometrical interpretation has only been developed for gravitation. Or in other words which feature distinguishes gravitation from the other three fundamental interactions? The answer lies in the "gravitational charge", the mass. It is a common observation that the gravitational mass


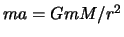





It is important to note that this behaviour distinguishes gravity from the other fundamental interactions which are associated with different types of charges, such as electric charge in the case of electromagnetic interaction. It is not obvious how and whether it is possible to obtain similar geometric formulations for the electromagnetic, weak and strong interaction. The unification of these three fundamental forces with gravity in the framework of quantum theory is one of the important areas of ongoing research.