These movies show simulations of isolated disk galaxies under the influence of “feedback” from massive, young stars. In the absence of such feedback, the gas in the galaxies would cool rapidly (in a time much shorter than a single orbital time), collapsing into dense ‘nuggets’ that run away to higher and higher densities until it eventually forms stars. In a single dynamical time, almost all of the gas would be turned into stars. In contrast, observations suggest that star formation is ~100 times slower than this rate. Moreover, a single cloud only turns a few percent of its total mass into stars, over its entire lifetime. This owes to the effect of massive stars on their surroundings. These effects include:
- Radiation pressure: Light (mostly from the youngest stars) scatters off gas and dust in the galaxy. Each time a photon scatters or is absorbed, it imparts some of its momentum to that gas, “pushing” away the gas and dust. This does not “heat up” the gas, but can impart an enormous amount of momentum.
- Stellar winds: Young stars blow winds off their surface that can have velocities as large as ~1000 km/s. This shocks and provides a large amount of thermal energy to heat the gas. Older stars blow “slow” winds at just ~10 km/s, but the total mass recycled into the ISM can be very large, ~30% of the original mass in stars.
- Photo-Ionization: The light from the stars also ionizes gas, heating it up to ~10^4 K. These ionized ‘bubbles’ can push on the gas significantly in very low-mass galaxies (where the corresponding velocities of the gas are comparable to the disk orbital velocities). It can also destroy molecules, a critical ingredient for the next generation of star formation.
- Supernovae: After a few million years, massive stars begin to explode as supernovae. Each such event imparts a large energy to the nearby ISM. Many “overlapping” events can build up huge hot bubbles of gas that generate pressures sufficient to “blow out” of the disk and vent material into the intergalactic medium.
To see what happens when these galaxies collide with one another in galaxy-galaxy mergers, click here.
This movie shows the gas in single dwarf galaxy (similar to the Small Magellanic Cloud), evolving for a few orbital periods. The color scale (above left) shows gas temperature, from <100 K molecular gas (blue) to >10^6 K ‘hot’ gas (yellow). Brightness shows the projected gas surface density, scaled logarithmically. Giant molecular clouds (GMCs) continuously form, and begin to form stars, but are quickly destroyed by feedback from young massive stars. In this case, a combination of radiation pressure ‘pushing’ on the gas and photo-ionization clears initial ‘holes’ and disrupts the clouds in just a couple million years. Then, supernovae begin to explode, inflating the large bubbles of hot gas that can be seen to grow and vent super-winds out of the disk. The mass in these winds can be ~10-20 times that turning into stars.
The picture at (right) shows a mock three-color image of what this galaxy would look like in visible light wavelengths. The ‘patchy’ gas distribution and star formation driven by feedback gives rise to the characteristic ‘irregular’ morphology observed in dwarf galaxies.
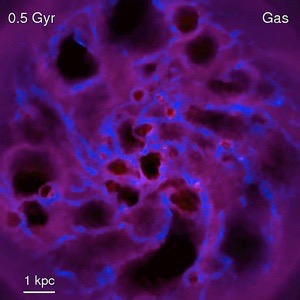
SMC - Radiation Pressure Alone
This simulation shows the same galaxy, but evolving without including the effects of supernovae ‘heating up’ the gas. Radiation pressure still dissociates molecular clouds shortly after they form, and regulates star formation in the disk, but there is a marked absence of ‘hot’ gas filling the ISM (and, in this case, that makes a dramatic difference to the galaxy winds as well).
This movie shows the same properties as the ‘SMC’ movie above. In this case, however, the initial galaxy model represents an extremely massive, high redshift starburst disk. Stars form at ~200 solar masses per year (200 times as fast as the Milky Way), driving explosive outflows from the disk. Because the gas in this disk is at extremely high densities, the thermal energy deposited by supernovae is radiated away almost immediately -- the ‘work’ done by feedback is dominated by the effects of radiation pressure, with huge amounts of starlight scattering off dust and gas. Explosive winds are driven, with large absolute masses, but relative to the star formation rate, they are about equal in magnitude. The characteristic ‘clumpy’ morphology observed in massive high-redshift disks arises because the disk is violently gravitationally unstable. However, many clumps are short-lived, continuously re-forming.
The picture at (right) again shows a mock three-color image. In this case, the instabilities (as well as dark patches obscured by dust) lead to a morphology dominated by a few bright clumps.
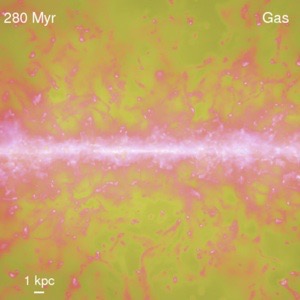
High-Redshift Starburst (Alternative)
This galaxy is similar to the one above in most respects, but is initialized with a somewhat different structure and mass in the galaxy halo and gaseous disk, as well as different feedback strength. As a result, the clumps are smaller and not so smooth, and tend to be shorter-lived. The winds driven from this galaxy are especially violent.
In this case, the initial galaxy is built to closely resemble the Milky Way. The same processes as in both cases above occur, with dense clouds continuously forming, and then dissolving under the influence of feedback, but on a (fractionally) much smaller scale. This happens because the gas fraction of the disk is much lower in this case than in either of the cases above. For this reason, the gas forms a more ordered ‘spiral’ pattern which eventually develops into a central ‘barred spiral’ typical of massive disk galaxies seen at low redshift. Because the star formation rate is quite low for a galaxy of this mass (about ~1 solar mass per year), the winds are weak, and almost entirely composed of ‘hot’ gas venting out of the disk.
The pictures at (right) again show a mock three-color image (face-on and edge-on). The spiral arms are clearly visible as dust lanes -- their global morphology comes from gravitational instabilities in the stars (which drag the gas with it), but their filamentary, irregular edges are the result of feedback from young stars. Young clusters of stars are visible as blue knots.