Galaxy formation in the reionization era
Understanding the formation of galaxies in the first billion years of the Universe is an important question in galaxy formation. Moreover, it is crucial for understanding the process of cosmic reionization, an active field in modern cosmology studying the phase transition when the hydrogen in our Universe changed from neutral to fully ionized.
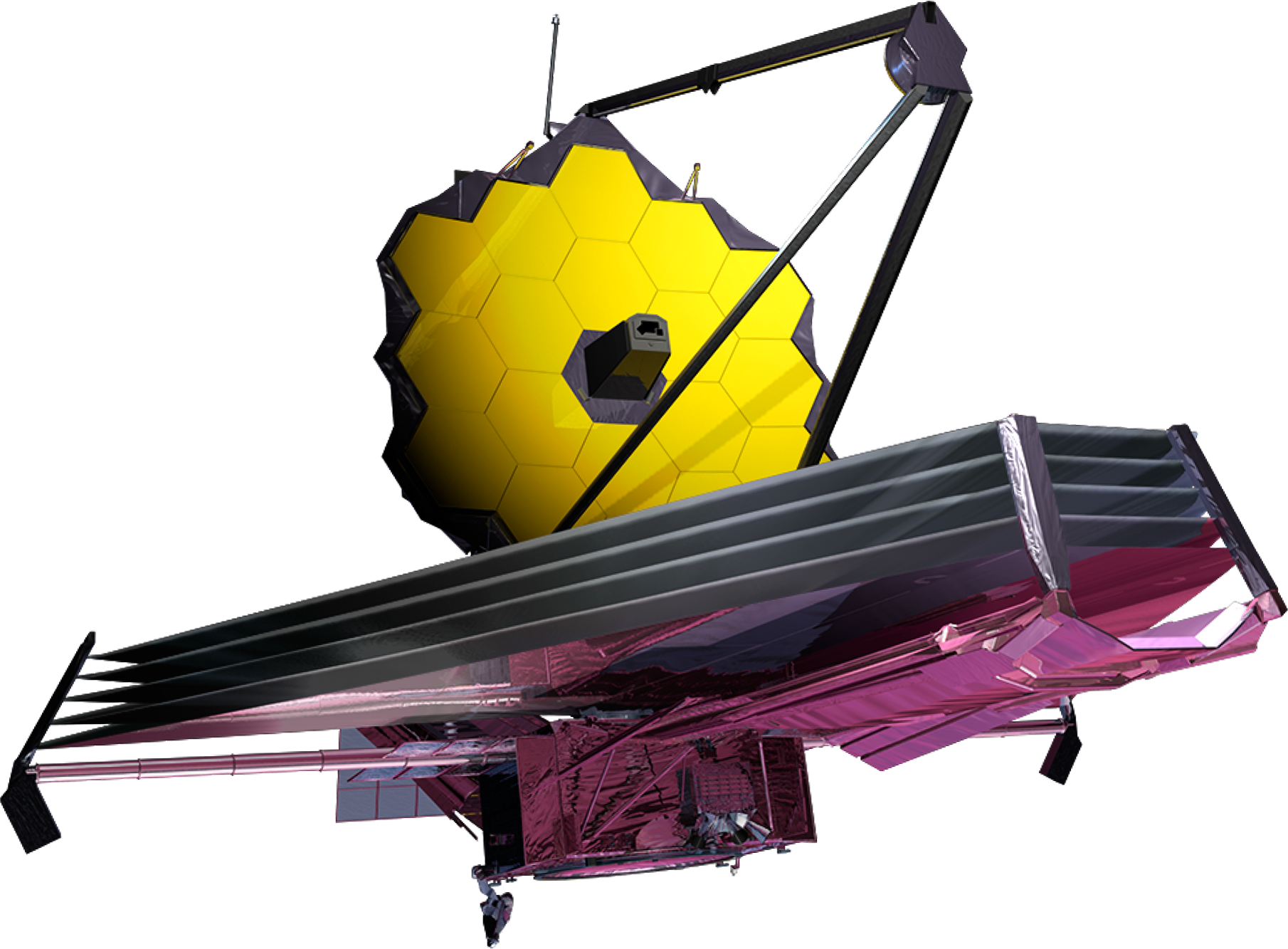
This will be the most rapidly evolving field in astronomy in the next 5-10 years: one major goal of the Hubble Space Telescope (HST), the James Webb Space Telescope (JWST), Roman Space Telescope (RST; previously WFIRST), the Atacama Large Millimeter Array (ALMA), intensity mapping experiments, and 30 m-class ground-based telescopes (TMT, GMT, and ELT) is to deliver a tremendous amount of high-quality data of galaxies at z ≳ 5 with unprecedented details in the next few decades.
FIRE in the high-redshift Universe
I initiate and develop the FIRE in the high-redshift Universe project, consisting of a suite of 38 high-resolution cosmological zoom-in simulations of galaxies in the reionization era (z ≳ 5), taking advantage of the explicitly resolve multi-phase ISM, star formation, and feedback models from the FIRE project. These simulations contain halos in Mhalo ~ 108-1012.5M⊙ at z ~ 5-15 at mass reloluion mb ~ 100-7000M⊙ and spatial resolution ≲ 1 pc in the densest gas. They allow us to attack a broad range of important questions about high-redshift galaxies:
- What are the star formation and metal enrichment histories of z ≳ 5 galaxies? How do the M*–Mhalo and M*–metallicity relations evolve at these redshifts?
- How do the stellar mass functions, luminosity functions, and star formation rate densities evolve at z ≳ 5?
- Are z ≳ 5 galaxies characterized by clumpy morphology and turbulent ISM? When do the first disks form? What are the possible observational biases in probing the properties of high-redshift galaxies?
- What are the Lyman-continuum photon escape fractions from z ≳ 5 galaxies? How much do they contribute to cosmic reionization? Can we indirecty measure the escape fractions using nebular emission lines?
- How do the Lyman-alpha properties depend on galaxy mass and redshift? Are there systematic uncertainties of using Lyman-alpha galaxies at z ≳ 5 to probe the reionization history?
- How much dust is there in z ≳ 5 galaxies? What fraction of light is attenuated? Is dust hotter at higher redshift?
- And many more...
These simulations have fostered 15 submitted/published paper to date and attracted broad interests from the community. Some most important results are highlighted below.
A challenge to galaxy formation theory
In particular, z ≳ 5 is when galaxies are most gas-rich, bursty, and chaotic, while independent simulation groups produce wildly diverging mass, morphology, kinematics, and other galaxy properties at these redshifts, due to their different ISM, star formation, and feedback models. This is a huge challenge to galaxy formation theory:
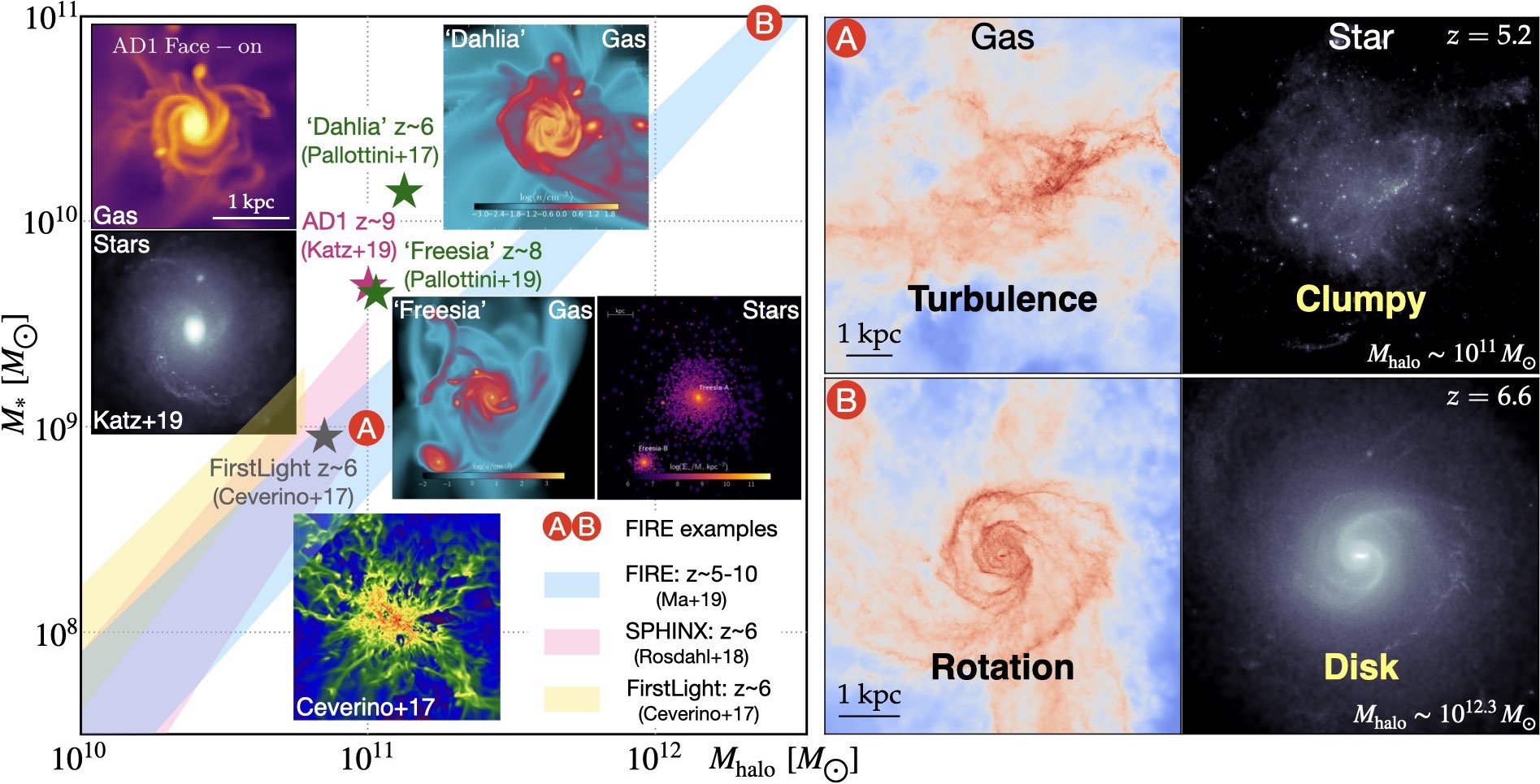
Figure: A compilation of cosmological simulations of galaxies at z ≳ 5 in the literature, showing in the M*–Mhalo relation. At similar Mhalo, FIRE predicts clumpy, turbulent ISM with little rotation, while some groups produce disk galaxies with superdense bulges and 3–10 times higher M*. FIRE makes disks only in M* ≳ 1011M⊙ galaxies.
Fortunately, with JWST, ALMA, and RST over the next decade, and 30 m-class telescopes thereafter, we will expect vast high-quality data of z ≳ 5 galaxies with unprecedented details. Confronting simulations with these high-z data hence offers a novel but powerful way for constraining galaxy formation physics.
Galaxy scaling relations & stellar mass/luminosity functions
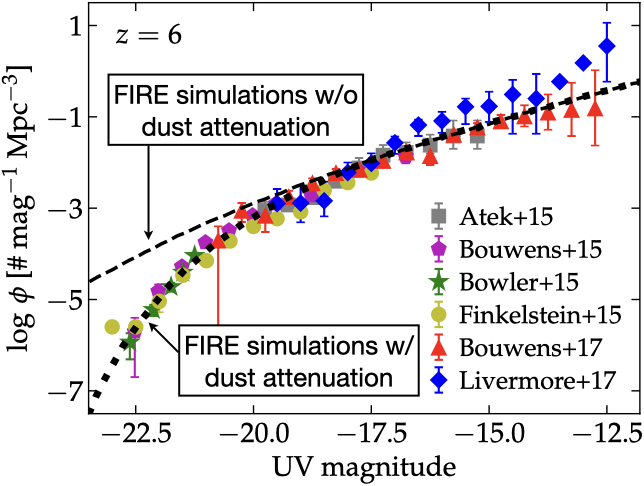
Figure: The galaxy UVLFs at z ~ 6. Our predictions agree well with observations when dust attenuation is included at the bright end (from Ma et al. 2018a).
In Ma et al. (2018a, MNRAS, 478, 1694), we predict the galaxy scaling relations at z = 5‒12, including the stellar mass‒halo mass relation (§3.2), SFR(Mhalo, z) and SFR(M*, z) relations (§3.3), multi-band magnitude‒halo/stellar mass relation, and the inverse halo/stellar mass‒magnitude relation (§3.4).
We further predict the stellar mass functions (SMFs; §4), multi-band luminosity functions (LFs; §5, §6.2), and cosmic star formation rate (CSFR) and stellar mass density (SMD; §6.1) at these redshifts. We make these predictions public.
Data release: We previde the machine-readable format of our predicted SMFs and multi-band LFs (click here). The files include (1) the SMFs and LFs at rest-frame UV, B band, and J band for intrinsic stellar continuum (i.e. without dust attenuation and line emission) derived from the simulated catalog (SMF_sim_zxx.txt, LF_UV_sim_zxx.txt, LF_B_sim_zxx.txt, and LF_J_sim_zxx.txt, where xx represents the redshift in two digits), (2) model SMFs and LFs obtained by convolving the halo mass functions with the best-fit scaling relations (SMF_model_zxx.txt, LF_UV_model_zxx.txt, LF_B_model_zxx.txt, and LF_J_model_zxx.txt), and (3) UVLFs after accounting for dust attenuation (LF_UV_red_zxx.txt).
Morphologies and sizes of high-redshift galaxies
In Ma et al. (2018b, MNRAS, 477, 219), we investigate the morphologies and sizes of galaxies in the reionization era, using the FIRE in the high-redshift Universe simulations. Like shown in the movie and figure below, we argue that the small, point-source like galaxies detected in the Hubble Frontier Fields (HFFs) are possibly individual star-forming regions, given the shallow detection limit of the HFFs survey.
These clumps are likely associated with young star clusters/complexes, which tend to form very efficiently in high-redshift galaxies (see details here).
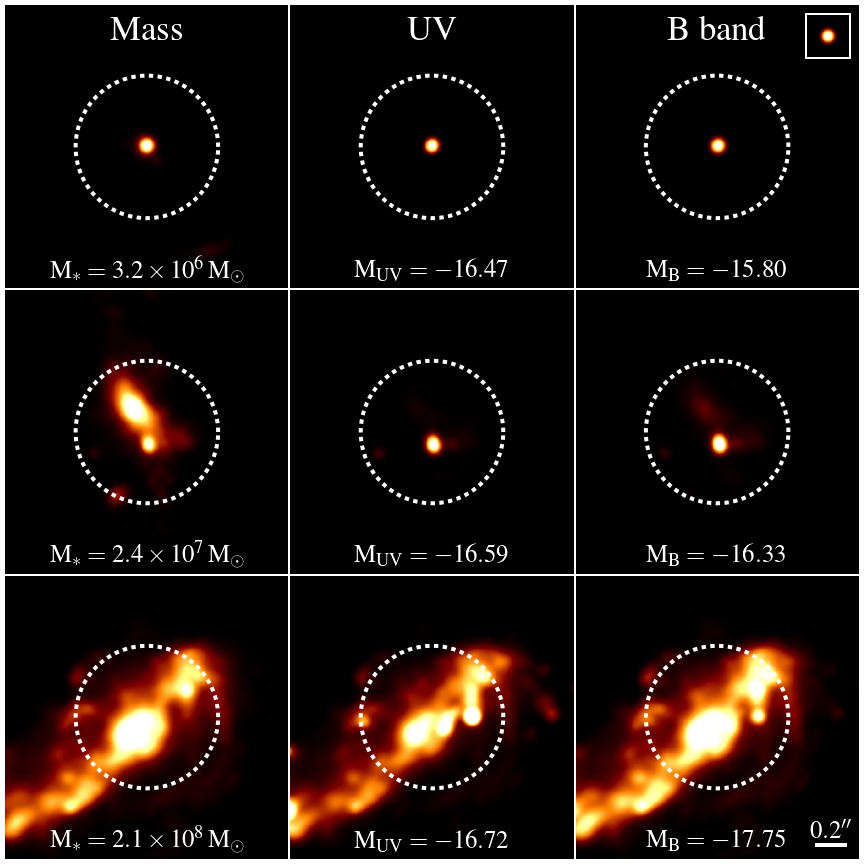
Movie: The rest-frame UV image (right) at varying detection limit. At limited depths, one can only see the brightest clumps but miss everything else. Figure: Stellar mass (left), rest-frame UV (middle), and B-band (right) images for three galaxies at MUV ~ -16.5. Their UV images are dominated by small bright clumps where stars formed recently (from Ma et al. 2018b).
Dust attenuation, emission & temperatures in high-z galaxies
In Ma et al. (2019, MNRAS, 487, 1844), we run the publicly available dust Monte Carlo radiative transfer code SKIRT on the entire FIRE in the high-redshift Universe simulation sample. By this means, we study dust attenuation, dust emission, and dust temperatures in galaxies at z ≥ 5. In particular, we emphasize that dust temperature generally increases with redshift, which must be taken into account when converting single-wavelength fluxes to total dust luminosities for recent ALMA observations.
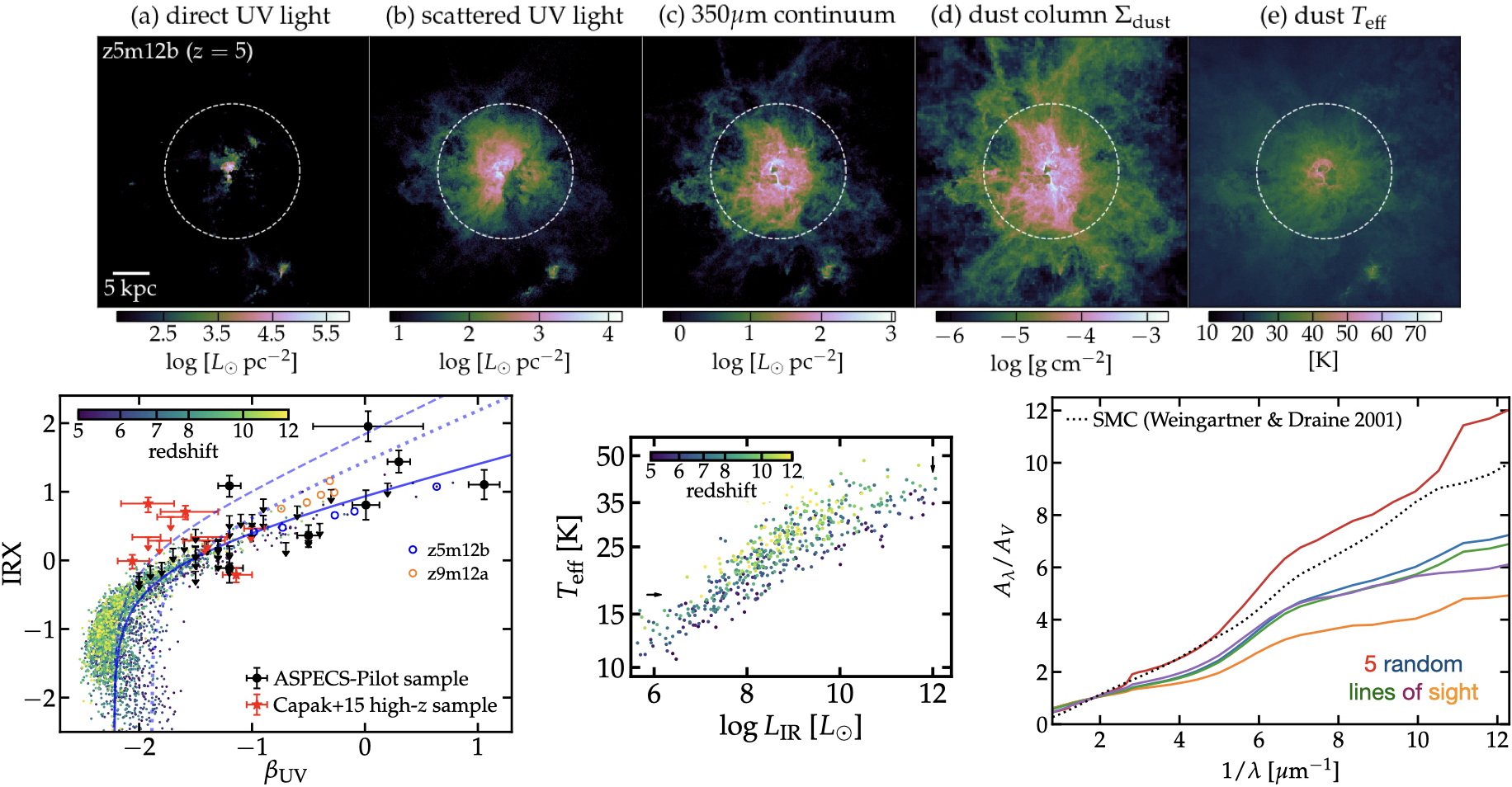
Figure: Top: Rest frame UV (a and b), far-IR (c), dust mass (d), and dust temperature (e) in a galaxy of stellar mass M* ~ 1010.5M⊙ at z ~ 5. Bottom left: IRX-β relation of z ≥ 5 galaxies. Bottom center: Effective dust temperature as a function of total dust luminosity and redshift (color). Bottom right: Average attenuation curve along 5 random sightlines.
Back to research